Carbon Capture Technologies Transforming Harmful Waste into Energy
As the world moves toward net-zero greenhouse gas emissions, capturing carbon dioxide from its source is becoming increasingly important. It can help make the process of phasing out fossil fuels possible.
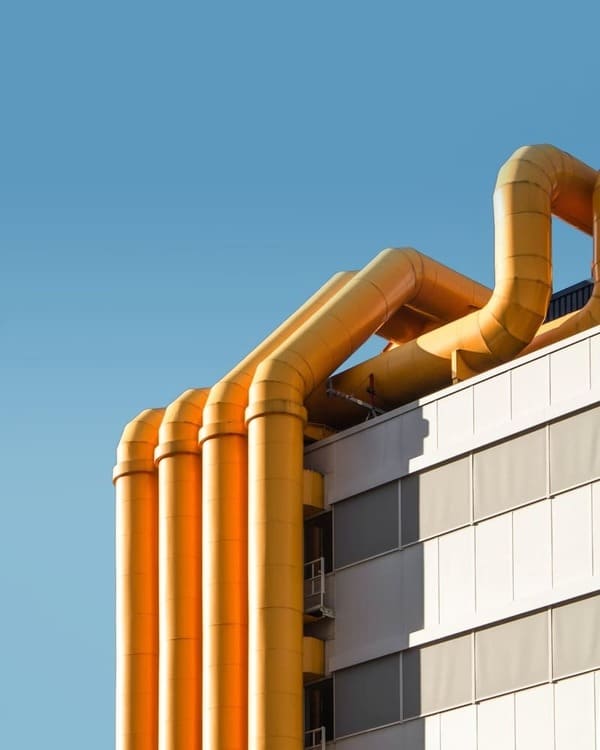
To do that, researchers need to find technologies for a wide range of emission sources. They also need to make them cheaper, more efficient, and easier to deploy on a commercial scale.
Contents
Chemical Solvents
For a carbon-capture technology to be effective, it must remove carbon dioxide at high pressure. This can be difficult, and it requires energy to do so. Ultimately, the CO2 must be stored underground.
One solution is to use a chemical solvent to convert industrial gas into pure CO2. These liquid amines have a strong affinity for carbon molecules, but they are expensive and toxic.
However, new, less-expensive solvents have been developed to overcome these drawbacks. These include mixed amine solvents, cyclic ammonia-based solvents, biphasic solvents, and ionic liquid-based solvents.
Many of these new, low-cost solvents also have superior cyclic loading capacity, a critical factor in PCC efficiency. For example, a biphasic solvent may require only 50% more regeneration energy than MEA, while an amino acid-based solvent might be three times more efficient in the initial conversion of CO2 to gas.
These new solvents also feature internal hydrogen bonding, which helps to reduce the number of interactions between molecules. This means fewer hydrogen atoms have to be used overall, thus lowering the cost of the process.
This research demonstrates that the technology can be economically and environmentally viable in the future, especially as coal-fired power plants are phased out and renewable energies become increasingly popular. Moreover, these new solvents can be deployed to treat a wide range of gasses that are now being emitted by a growing array of point sources.
CCUS will continue to evolve as energy systems shift towards cleaner, renewable fuels. Nevertheless, there will still be a need for treatment of concentrated exhaust streams from existing point sources of fossil-fuel-fired electricity generation and cement and steel factories that will continue to emit these gasses.
As a result, reducing or eliminating CO2 emissions from fossil fuel-fired power plants and other industries will require technological adaptations to existing CCUS processes. These will be important to ensure a transition to a more sustainable, clean energy future.
Physical Solvents
Physical solvents are a key part of carbon capture technologies that transform harmful waste into energy. These processes use a combination of chemical and physical properties to remove carbon dioxide from gasses that are emitted by industry. The most mature and commercially deployed carbon capture technologies rely on these liquid absorption processes.
These processes remove CO2 and acid gasses from natural gas streams with high efficiencies and are capable of being scaled up for point-source applications such as coal and gas power plants, cement kilns, and steel furnaces. In addition to their thermodynamic efficiency, these processes have the lowest total costs of capture and separation (TCOs), requiring the least investment per tonne of captured CO2.
Selexol Process
The most widely used physical solvent process is the Selexol process, which employs dimethyl ethers of polyethylene glycol [DPEG or Genosorb 1753] as its sorbent. The solvent is chemically and thermally stable, has a low vapor pressure, and limits its losses to the treated gas. It also allows for the treatment of a wide range of acid compositions and can be operated under a variety of flow schemes to achieve the desired product specifications.
Rectisol Process
Another common physical solvent process is the Rectisol process, which uses methanol to remove hydrogen sulfide and carbonyl sulfide and to produce clean gas at acid compositions down to ppm sulfur. The process can be adapted to handle a variety of gas compositions and treating pressures and is a flexible and economic alternative to other processes.
In this process, a chemically reacting alkanol amine is blended with water and a physical solvent called sulfolane (tetrahydrothiophene dioxide). The actual formulation of the solvent is customized for each application. The process is effective in removing carbonyl sulfide, mercaptan derivatives, and other organic sulfur compounds to stringent total sulfur specifications.
The process is also able to remove H2S, CO2, and heavy hydrocarbons with a partial pressure of 50 psi or higher, but these heavy hydrocarbons are lost from the gas stream, which cannot be economically recovered. This is why the physical solvent process is not generally considered a good choice for rich gas streams that have significant quantities of C3+ hydrocarbons.
Membranes
Gas separation membranes are a key component in carbon capture processes. These membranes use a chemical reaction to separate carbon dioxide from other gasses. They are also used in the production of hydrogen, a clean fuel that can be used in hybrid vehicles.
There are a variety of different membranes that can be used in this process. Typically, they have a high permeability and high selectivity for the gasses that they separate. The permeability of the membrane allows the gas to flow through it quickly, while the selectivity allows the CO2 to be captured.
For this reason, membranes have the potential to be an energy-efficient solution for carbon capture. The main challenge is to find the right balance between permeability and selectivity.
To accomplish this, a range of membrane performances and operating conditions must be evaluated. This can be done through engineering parametric studies that look at the impact of material performances and operating conditions on the CO2 recovery ratio.
This will allow the developer to determine the best combination of pressure ratio, stage cut, and membrane surface area to achieve the desired process separation performance. This is critical since each of these parameters can make a difference in CO2 purity and CO2 recovery.
Typical separations for single-stage processes include a relatively low module pressure ratio, a very low stage cut, and relatively high membrane permeance ( 3 m3(STP)/(m2 h bar)). In a two-stage process, the membrane surface area must be larger to ensure adequate permeate production rates.
Solid Sorbents
Carbon capture technologies are used to transform harmful waste into energy, by capturing CO2 emissions from coal and natural gas power plants and industrial processes that produce cement, chemicals, or metals. The process also has the potential to reduce reliance on fossil fuels.
The most common carbon capture technology involves a liquid that is able to chemically remove CO2 from a gaseous stream before it can be released into the air. This is a promising approach because it can be implemented at existing plants. However, it’s also a costly approach and can be difficult to implement.
Another form of carbon capture is referred to as pre-combustion. This is a process that essentially takes carbon dioxide out of fossil fuels before they are burned, using steam and oxygen to convert the fuel into a mix of hydrogen and CO2 called syngas. This can then be converted to a more conventional form of energy like electricity, or converted into fuel for use in a vehicle, such as natural gas or propane.
Several companies, including Global Thermostat, Climeworks, and Carbon Engineering, are developing these systems. These processes involve the adsorption of CO2 onto solid sorbents that are either amine (or ammonium)-based or made from other structured materials.
A number of factors can affect the efficiency of a solid sorbent process, such as the amount of CO2 captured, the temperature of the sorbent material, and the rate of desorption of the sorbent. These factors all affect the energy cost of the adsorption-based process, and it is important to consider these in the analysis.
A solid sorbent-based process requires substantial amounts of water. Typically, water losses in the adsorption-based approach can be as high as 1.6 Mt/y. This could vary depending on the ambient humidity at the capture site and the sorption rate.
Direct air capture is a relatively new technique that is often touted as a way to draw CO2 out of the atmosphere, especially if a carbon tax or cap-and-trade program was implemented. Although it can be more expensive than planting trees, many scientists say it is an important strategy for addressing climate change.
Final Word
Carbon capture technologies offer a promising solution to the global climate crisis.
By capturing waste and turning it into energy, these technologies are transforming harmful emissions into useful resources for power generation, reducing the amount of carbon dioxide being released into the atmosphere.
This technology can be used in various industries ranging from power plants to industrial processes. Carbon capture technologies are also relatively cost-effective, making them a viable option for companies and governments seeking to mitigate their carbon footprint.
In the coming years, these technologies will become increasingly important as we continue to face the challenges of global warming.
With the right investments in research and development, carbon capture can help us achieve a sustainable future while protecting our environment.